Assembly Line Robotics: The Ultimate Guide to Automation in Manufacturing
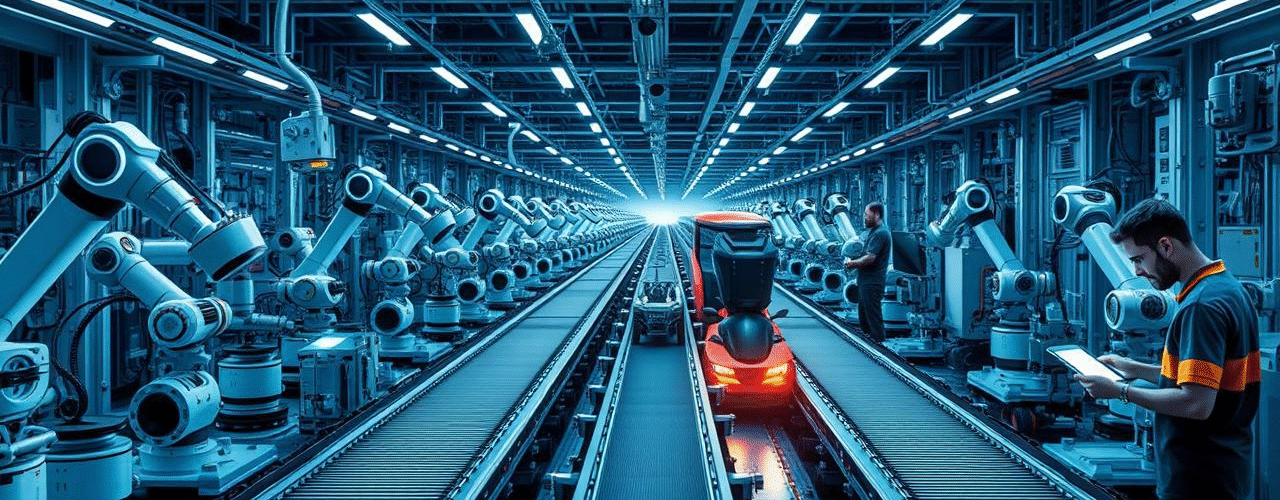
Have you ever wondered how your smartphone, car, or even your morning coffee maker comes together so perfectly? Behind the scenes of modern manufacturing lies a technological marvel that’s revolutionizing how products are made: assembly line robotics. These tireless machines are transforming factories worldwide, creating unprecedented levels of efficiency and precision that were unimaginable just decades ago. Assembly line robotics represents the cutting edge of industrial automation, combining artificial intelligence, advanced mechanics, and sophisticated programming to create the products we rely on daily.
This comprehensive guide explores everything you need to know about assembly line robotics—from their history and evolution to the latest innovations shaping the future of manufacturing. Whether you’re an industry professional looking to upgrade your production line, a business owner considering automation, or simply curious about how modern factories operate, this guide delivers valuable insights into this fascinating technology.
Table of Contents
The Evolution of Assembly Line Robotics
From Ford’s Innovation to Modern Automation
The concept of the assembly line itself dates back to 1913 when Henry Ford revolutionized manufacturing with his moving assembly line. This innovation reduced the time to build a Model T from 12 hours to just 93 minutes. However, the first true industrial robot wouldn’t appear until 1961 when General Motors deployed the Unimate—a robotic arm that handled die casting and spot welding.
In those early days, robots were massive, dangerous machines that performed simple, repetitive tasks. They required extensive safety barriers and had limited capabilities compared to today’s standards. The transformation since then has been remarkable.
Key Milestones in Assembly Line Robotics
- 1961: Unimate becomes the first industrial robot deployed in a factory
- 1970s: The introduction of microprocessors enables more sophisticated robot programming
- 1980s: Vision systems begin allowing robots to “see” their environment
- 1990s: Networked robots create the first truly integrated production systems
- 2000s: Collaborative robots (cobots) emerge, designed to work safely alongside humans
- 2010s: AI and machine learning enable adaptive robots that can learn from experience
- 2020s: Autonomous mobile robots (AMRs) bring flexibility and intelligence to the factory floor
Today’s assembly line robots are sophisticated machines that combine multiple technologies—vision systems, AI algorithms, precision mechanics, and advanced sensors—to create flexible manufacturing systems that can handle complex tasks with minimal human intervention.
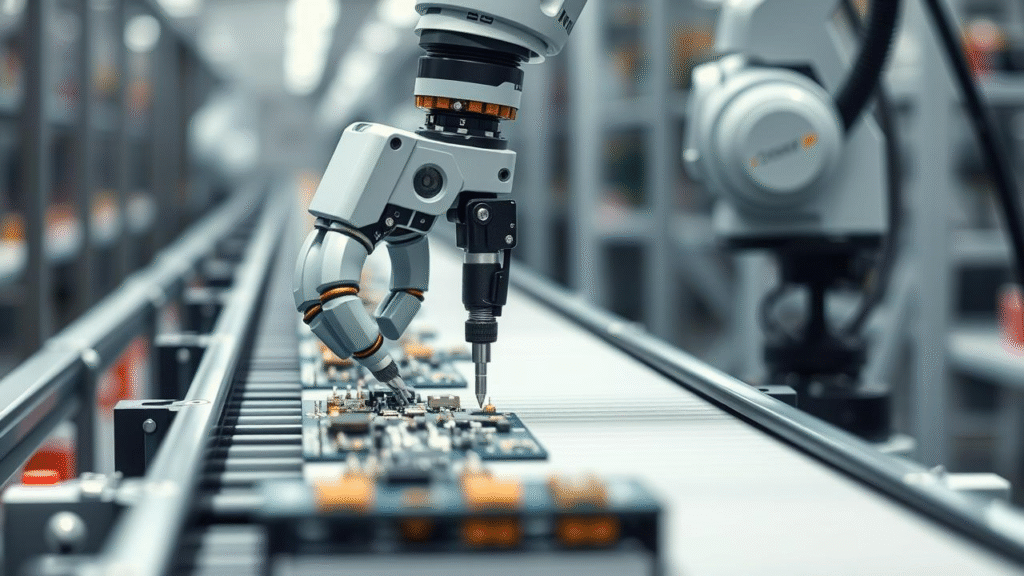
Types of Assembly Line Robots
Modern manufacturing employs several distinct robotic systems, each specialized for particular assembly line functions.
Articulated Robots
These are the most common industrial robots, featuring a mechanical arm with multiple joints resembling a human arm. With typically 4-6 axes of motion, articulated robots offer exceptional flexibility for complex movements. They excel at tasks requiring dexterity such as welding, painting, assembly, pick-and-place operations, and material handling.
The FANUC R-2000iB series represents a classic example, capable of handling payloads up to 250kg with a reach of nearly 3 meters—all while maintaining repeatability within 0.2mm.
SCARA Robots
Selective Compliance Assembly Robot Arms (SCARA) are specialized for lateral movements, making them ideal for assembly operations requiring precise horizontal movements but limited vertical capability. Their design provides rigidity for vertical loads while allowing flexibility in the horizontal plane.
SCARA robots perform exceptionally well in electronics manufacturing, particularly in circuit board assembly where components must be placed with microscopic precision. Their speed and accuracy make them perfect for high-volume production where cycle times are critical.
Delta Robots
When speed is paramount, delta robots reign supreme. These spider-like machines feature parallel arms connected to a common base, allowing for incredibly fast movement. Some delta robots can achieve more than 300 picks per minute—far faster than human capabilities.
Food packaging, pharmaceutical sorting, and other light-payload, high-speed applications benefit from delta robots’ unmatched velocity and precision. Their unique design minimizes moving mass, enabling the remarkable acceleration that makes them ideal for pick-and-place operations.
Collaborative Robots (Cobots)
Unlike traditional industrial robots that operate inside safety cages, collaborative robots work directly alongside human workers. Built with rounded edges, force-limitation technology, and sophisticated sensors, cobots stop immediately when they encounter unexpected resistance, making them safe for human interaction.
Cobots excel in applications where complete automation isn’t practical or where human judgment complements robotic precision. Assembly tasks requiring both dexterity and decision-making benefit from this human-robot collaboration. The Universal Robots UR series exemplifies this category, offering payloads from 3-16kg with built-in safety features.
Autonomous Mobile Robots (AMRs)
The newest category transforming assembly lines, AMRs navigate independently through factory environments, transporting materials between workstations without fixed paths. Unlike traditional automated guided vehicles (AGVs) that follow predefined routes, AMRs use sensors and AI to map their surroundings and plan optimal paths.
This flexibility eliminates the need for expensive infrastructure modifications and allows for dynamic reconfiguration of manufacturing processes. Companies like Amazon Robotics have deployed thousands of AMRs in fulfillment centers, dramatically increasing throughput while reducing labor costs.
Core Components of Assembly Line Robotics
Modern assembly line robots comprise several sophisticated systems working in harmony:
Mechanical Systems
The physical structure of a robot determines its capabilities and limitations. Key mechanical elements include:
- Actuators: Convert energy (typically electrical) into physical movement
- Joints: Allow for specific types of motion (rotational, prismatic, etc.)
- End effectors: The “hands” of the robot that interact directly with workpieces
- Transmission systems: Gears, belts, and other components that transfer power through the robot
Advanced materials science has enabled lighter, stronger robot structures that maintain rigidity while reducing energy consumption. Carbon fiber components, high-strength aluminum alloys, and specialized composites contribute to modern robots’ impressive strength-to-weight ratios.
Control Systems
The “brain” of an assembly line robot includes:
- Controllers: Industrial computers that execute programmed instructions
- Servo drives: Precision motor controllers that maintain exact positioning
- Programming interfaces: Software that allows humans to instruct robot behavior
- Safety systems: Emergency stops and monitoring systems that prevent accidents
Modern robot controllers often integrate with factory-wide Manufacturing Execution Systems (MES) and Enterprise Resource Planning (ERP) systems, creating seamless information flow throughout the production process.
Sensory Systems
To interact effectively with their environment, robots rely on various sensing technologies:
- Vision systems: Cameras with specialized software that recognize objects and positions
- Force/torque sensors: Measure pressures and forces during operations
- Proximity sensors: Detect nearby objects without physical contact
- Tactile sensors: Provide feedback about physical contact with objects
The integration of multiple sensor types creates robots that can adapt to variations in parts, positions, and environments—a capability essential for modern flexible manufacturing.
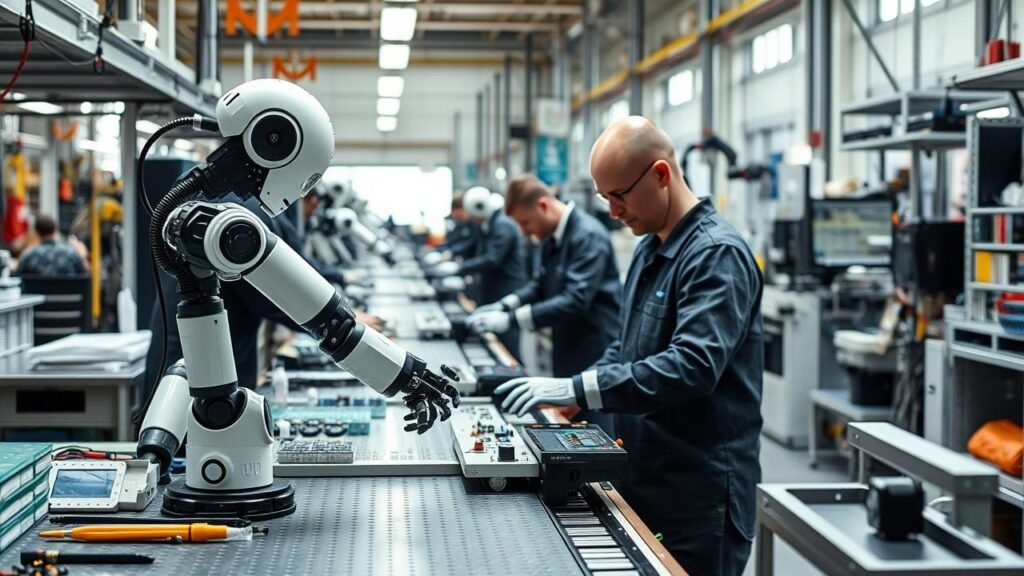
Benefits of Assembly Line Robotics
Enhanced Productivity and Efficiency
The numbers tell a compelling story: robots dramatically increase manufacturing output. A typical industrial robot can work continuously 24/7, requiring only occasional maintenance downtime. This translates to production increases of 30-50% compared to manual processes, according to data from the International Federation of Robotics.
Beyond raw speed, robots maintain consistent performance throughout their operating period, eliminating the productivity decline that humans experience due to fatigue. This consistency creates predictable production rates that simplify planning and scheduling.
Superior Quality and Precision
Human workers, no matter how skilled, experience natural variations in performance. Robots, however, can repeat the same motion thousands of times with sub-millimeter precision. This exceptional repeatability translates directly to product quality.
Manufacturing operations utilizing robotic assembly typically report defect reductions of 20-40%, with some precision applications seeing even more dramatic improvements. For industries where precision is non-negotiable—medical devices, electronics, aerospace—robotic assembly provides the consistency necessary for high-reliability products.
Cost Reduction Over Time
While the initial investment in robotics is substantial—industrial robots typically cost between $50,000 and $200,000 per unit before installation—the long-term economics are compelling. When accounting for labor savings, quality improvements, reduced waste, and increased throughput, most robotic installations achieve return on investment within 2-3 years.
The economics become even more favorable as robot prices continue to decline while capabilities increase. Between 2010 and 2020, the average cost of industrial robots fell by approximately 50% when adjusted for capabilities, making automation accessible to smaller manufacturers.
Improved Worker Safety
Manufacturing environments present numerous hazards—heavy lifting, repetitive motion injuries, exposure to harmful substances, and dangerous machinery. Robots take on these risky tasks, dramatically reducing workplace injuries.
Companies implementing comprehensive robotic automation typically report workplace injury reductions of 50% or more. Beyond the human benefit, this translates to significant cost savings in workers’ compensation, lost productivity, and insurance premiums.
Enhanced Flexibility and Adaptability
Modern robots can switch between different products and processes much faster than reconfiguring human-operated assembly lines. Software-driven changeovers can happen in minutes rather than hours or days, enabling economical small-batch production that was previously impractical.
This flexibility allows manufacturers to respond quickly to market demands, reducing inventory requirements and enabling just-in-time production strategies. Some advanced facilities can economically produce batches as small as single units—true mass customization.
Implementation Challenges and Solutions
Integration with Existing Systems
Few manufacturers have the luxury of building factories from scratch. Most must integrate robotic systems with existing equipment, software, and processes. This integration presents significant challenges:
- Interfacing with legacy machinery that lacks modern communication capabilities
- Ensuring data consistency across disparate systems
- Maintaining production during transition periods
- Training existing staff on new technologies
Successful integration typically employs a phased approach, starting with standalone robotic cells that gradually connect to wider factory systems. Interface technologies like OPC UA (Open Platform Communications Unified Architecture) provide standardized communication protocols that bridge old and new systems.
Programming and Setup Complexity
Traditional robot programming required specialized knowledge of proprietary languages and complex coordinate systems. This created a significant skills barrier for many manufacturers.
Modern solutions address this challenge through:
- Offline programming software that allows simulation and testing before physical implementation
- Teach-by-demonstration systems where operators physically guide robots through desired movements
- Intuitive graphical interfaces that simplify programming for non-specialists
- Pre-programmed application packages for common tasks like palletizing or welding
These approaches significantly reduce implementation time while making robotics accessible to companies without specialized programming expertise.
Cost Considerations and ROI Calculations
The financial case for robotics must account for numerous factors beyond the obvious labor savings:
- Initial equipment costs (robots, end effectors, safety systems)
- Integration engineering and installation
- Training and workforce transition
- Ongoing maintenance and updates
- Energy consumption
- Production increases and quality improvements
Comprehensive ROI calculations typically show that medium to high-volume manufacturers achieve payback periods of 18-36 months. However, the financial equation depends heavily on application-specific factors including labor costs, precision requirements, and production volumes.
Workforce Adaptation and Training
Perhaps the most significant implementation challenge involves human factors. Existing workers often fear job displacement and may resist automation initiatives. Successful implementation requires thoughtful workforce transition strategies:
- Transparent communication about automation plans and timelines
- Retraining programs that help workers develop skills for operating and maintaining robotic systems
- Creating new roles that leverage human creativity and problem-solving alongside robotic precision
- Gradual implementation that allows for adjustment and learning
Companies that handle these human elements effectively typically see higher success rates and faster return on investment from their automation projects.
Industry Applications and Success Stories
Automotive Manufacturing
The automotive industry remains the largest user of industrial robots, deploying approximately 40% of all units sold globally. Modern car production exemplifies integrated robotics:
- Body shops utilize hundreds of welding robots that join metal components with submillimeter precision
- Painting robots achieve perfectly uniform finishes while reducing environmental impact from overspray
- Assembly operations combine heavy-duty robots for dashboard installation with collaborative robots for delicate wiring and trim work
Tesla’s Fremont factory represents the cutting edge of automotive automation, using more than 1,000 robots throughout its production process. This extensive automation helps Tesla produce vehicles with approximately 30% fewer labor hours than traditional manufacturers.
Electronics Manufacturing
Consumer electronics present unique challenges—delicate components, microscopic connections, and rapid product cycles. Robotics meets these challenges through:
- Ultra-precise SCARA robots that place components on circuit boards with accuracy to 0.01mm
- Vision-guided systems that inspect solder joints and component placement at speeds impossible for human workers
- Flexible assembly systems that can switch between different product variations in minutes
Foxconn, the world’s largest electronics contract manufacturer, has deployed more than 100,000 robots in its facilities. These “Foxbots” perform precision tasks while allowing the company to adapt quickly to changing product specifications from clients like Apple and Samsung.
Food and Beverage Production
Once limited to end-of-line packaging, robots now handle numerous food production tasks:
- Delta robots that pick and sort irregular food items at rates exceeding 200 pieces per minute
- Collaborative robots that work alongside humans for artisanal food production
- Specialized systems that maintain hygiene standards while handling unpackaged food products
Ocado, the online grocery specialist, demonstrates the potential of advanced robotics in food handling. Their automated warehouses feature thousands of robots that retrieve grocery items from a complex storage grid, enabling them to process 65,000 orders weekly with minimal human intervention.
Pharmaceutical Manufacturing
The pharmaceutical industry’s stringent quality requirements make it an ideal candidate for robotic assembly:
- Sterile environments where robots eliminate human contamination risks
- Precision dispensing of active ingredients with zero variance
- Complete traceability through integrated vision and tracking systems
- Rapid changeover between different medications to enable economical small-batch production
Amgen’s next-generation biomanufacturing facility in Singapore showcases pharmaceutical robotics at its best. Their highly automated processes enable production capacity increases of 80% while reducing operating costs by 60% compared to conventional facilities.
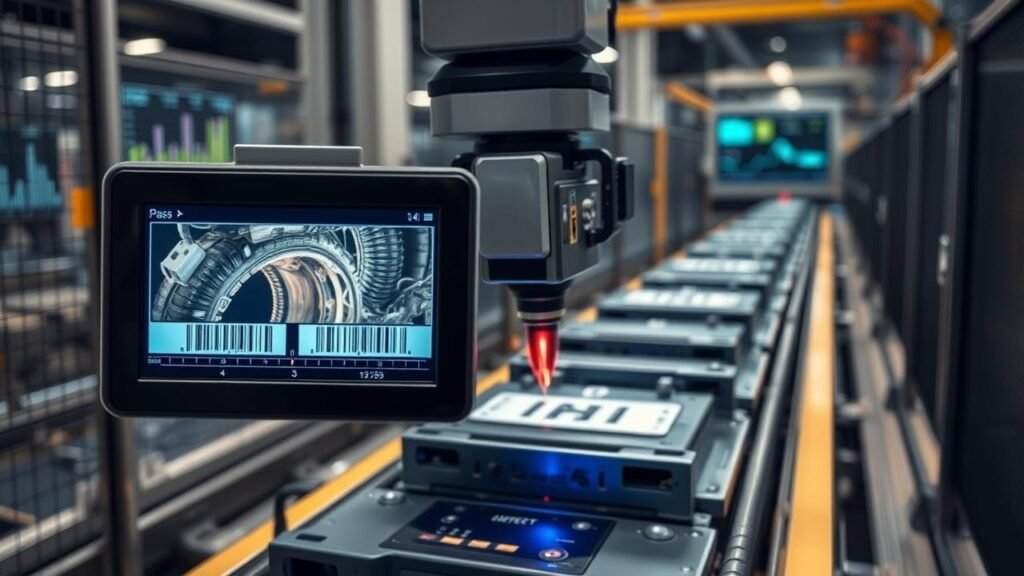
The Future of Assembly Line Robotics
AI and Machine Learning Integration
While today’s robots excel at programmed tasks, tomorrow’s systems will adapt and learn continuously:
- Self-optimizing robots that adjust their movements to achieve better results
- Vision systems that recognize previously unseen objects and determine appropriate handling
- Predictive maintenance algorithms that schedule service before failures occur
- Dynamic work allocation between robots and humans based on real-time conditions
These AI capabilities will create truly flexible manufacturing systems that require minimal human intervention while continuously improving their performance.
Advanced Human-Robot Collaboration
The strict separation between human and robot work zones is disappearing. Future factories will feature seamless collaboration:
- Augmented reality interfaces that allow humans to “see” robot intentions and status
- Natural language processing enabling verbal communication between workers and robots
- Haptic feedback systems that let humans “feel” what robots are experiencing
- Shared workspaces where multiple robots and humans cooperate on complex assemblies
This enhanced collaboration combines human creativity and problem-solving with robotic precision and endurance, creating manufacturing capabilities beyond what either could achieve alone.
Increased Flexibility and Mobility
Fixed installation robots are giving way to more adaptable systems:
- Mobile manipulation platforms that combine AMR mobility with articulated arm dexterity
- Quickly reconfigurable workcells that adapt to different products without physical rebuilding
- Modular robot designs that allow rapid function changes through end-effector swapping
- Wireless power and communication eliminating the need for fixed connections
These advances enable “pop-up manufacturing” where production can be established quickly in new locations to meet changing market demands or supply chain requirements.
Sustainable Manufacturing
Environmental considerations are driving new directions in robotics development:
- Energy-efficient designs that reduce power consumption through lightweight materials and regenerative braking
- Precision application systems that minimize material waste
- Robots specifically designed for disassembly and recycling operations
- Extended equipment lifespans through modular upgrades rather than complete replacement
These sustainability features align environmental responsibility with economic benefits, creating compelling value propositions for forward-thinking manufacturers.
Getting Started with Assembly Line Robotics
Assessment and Planning
The journey toward robotic assembly begins with thorough assessment:
- Process analysis: Identify operations suitable for automation based on repetition, precision requirements, ergonomic concerns, and quality challenges
- Data collection: Establish baseline metrics for current operations (cycle times, defect rates, labor costs)
- Prioritization: Rank potential automation projects based on ROI and implementation complexity
- Roadmap development: Create a phased implementation plan aligned with business objectives
This methodical approach ensures investments target the applications with greatest potential impact while managing implementation complexity.
Selecting the Right Technology Partners
The rapidly evolving robotics landscape makes partner selection crucial:
- System integrators: Firms specializing in implementing robotic solutions
- Robot manufacturers: Suppliers of the core robotic hardware
- Software providers: Companies offering programming, simulation, and management tools
- End-effector specialists: Developers of the application-specific “hands” that robots use
Effective partnerships often involve multiple specialists collaborating to create complete solutions tailored to specific manufacturing environments.
Pilot Projects and Scaling
Successful implementations typically follow a proven progression:
- Pilot project: Implement a single robotic cell for a well-defined application
- Performance validation: Thoroughly measure outcomes against predictions and expectations
- Process refinement: Optimize the solution based on initial learnings
- Strategic expansion: Apply successful patterns to additional applications throughout the facility
This measured approach minimizes risk while building internal expertise and confidence in robotic technology.
Building Internal Capabilities
Long-term success requires developing organizational capabilities:
- Technical training: Equipping maintenance staff with robot service skills
- Operational training: Preparing production teams to work effectively with automated systems
- Programming expertise: Developing internal ability to modify and optimize robot behavior
- Continuous improvement culture: Establishing processes to identify and implement ongoing enhancements
Organizations that develop these internal capabilities achieve significantly better long-term results from their automation investments.
Common Myths About Assembly Line Robotics
“Robots Will Replace All Human Workers”
Reality: While robots excel at repetitive, precise tasks, they lack human adaptability, creativity, and problem-solving abilities. Most successful implementations create human-machine teams that leverage the strengths of both. Studies by the World Economic Forum suggest that while robotics eliminates some roles, it creates others—often higher-skilled positions in programming, maintenance, and system optimization.
“Robotics Is Only for Large Manufacturers”
Reality: The emergence of affordable, easy-to-implement collaborative robots has made automation accessible to small and medium enterprises (SMEs). Leasing options, robotics-as-a-service models, and quick-deployment packages significantly reduce barriers to entry. Companies with as few as 20 employees are successfully implementing robotic assembly solutions.
“Implementation Is Too Disruptive”
Reality: Modern implementation approaches minimize disruption through careful planning and phased deployment. Many robotic systems can be installed and tested offline, then rapidly deployed during planned downtime. Some collaborative applications can be implemented with zero disruption to existing processes.
“Programming Requires Specialized Expertise”
Reality: While complex applications still benefit from specialist knowledge, many modern robots offer intuitive programming interfaces. Graphical programming environments, teach-by-demonstration capabilities, and pre-configured application packages make basic robot programming accessible to existing manufacturing staff with minimal training.
Conclusion
Assembly line robotics represents one of the most significant manufacturing advances since Ford’s original moving assembly line. From automotive plants to electronics factories, food processing facilities to pharmaceutical labs, robots are transforming how products are made—improving quality, enhancing safety, reducing costs, and enabling unprecedented flexibility.
As artificial intelligence, sensing technologies, and mechanical designs continue to advance, the capabilities of assembly line robots will expand further. The factories of tomorrow will feature intelligent, collaborative systems that combine the precision and endurance of robots with the creativity and adaptability of skilled human workers.
Whether you’re considering your first robotic implementation or expanding an existing automation program, understanding the technology landscape, implementation best practices, and potential challenges is essential for success. By taking a strategic, measured approach to robotic assembly, manufacturers of all sizes can realize the substantial benefits of this transformative technology.
References and Further Reading
Below are authoritative sources for additional information on assembly line robotics:
- International Federation of Robotics – World Robotics Report
- MIT Technology Review – “The State of Industrial Robotics”
- FANUC Robotics – Technical Documentation and Application Guides
- Universal Robots – Collaborative Robot Implementation Resources
- IEEE Robotics and Automation Society – Research Publications
- McKinsey Global Institute – “Automation, Employment, and Productivity”
- American Society of Mechanical Engineers – Manufacturing Automation Standards
- National Institute of Standards and Technology – Robotics Test Methods
- Robotics Industries Association – Safety Standards and Guidelines
- Manufacturing Engineering Magazine – Case Studies in Robotic Implementation